Introduction
The Cadomian Orogeny was part of the Pan-African Orogeny, occurring along the north-western margin of Gondwana (peri-Gondwana, Fig. 1.1) from the mid-Proterozoic to the earliest Cambrian (i.e. at the end of the Pan-African Orogeny.)
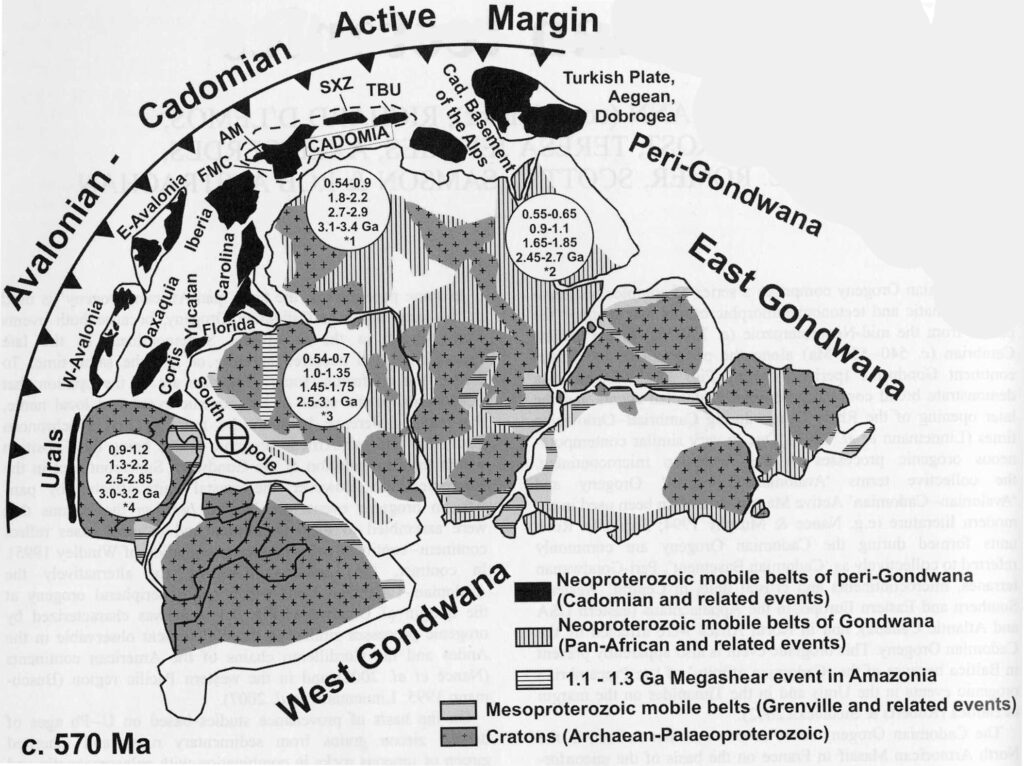
Several important geological areas in Europe belonged to Cadomia, including parts of the Bohemian Massif and of the Saxo-Thuringian Zone, the Moldanubian Zone, the Iberian Peninsula, the French Massif Central, the Massif des Maures, the Pyreneés and the Armorican Massif (Fig. 1.2. – not shown: Iberian Peninsula.) Unfortunately, most of these were overprinted by later orogenies, especially the Caledonian and Variscan orogenies. However, the northern part of the Armorican Massif remained mostly unscathed and thus provides an excellent insight into the Cadomian Orogeny – which is why it will be the focus of attention of this post.
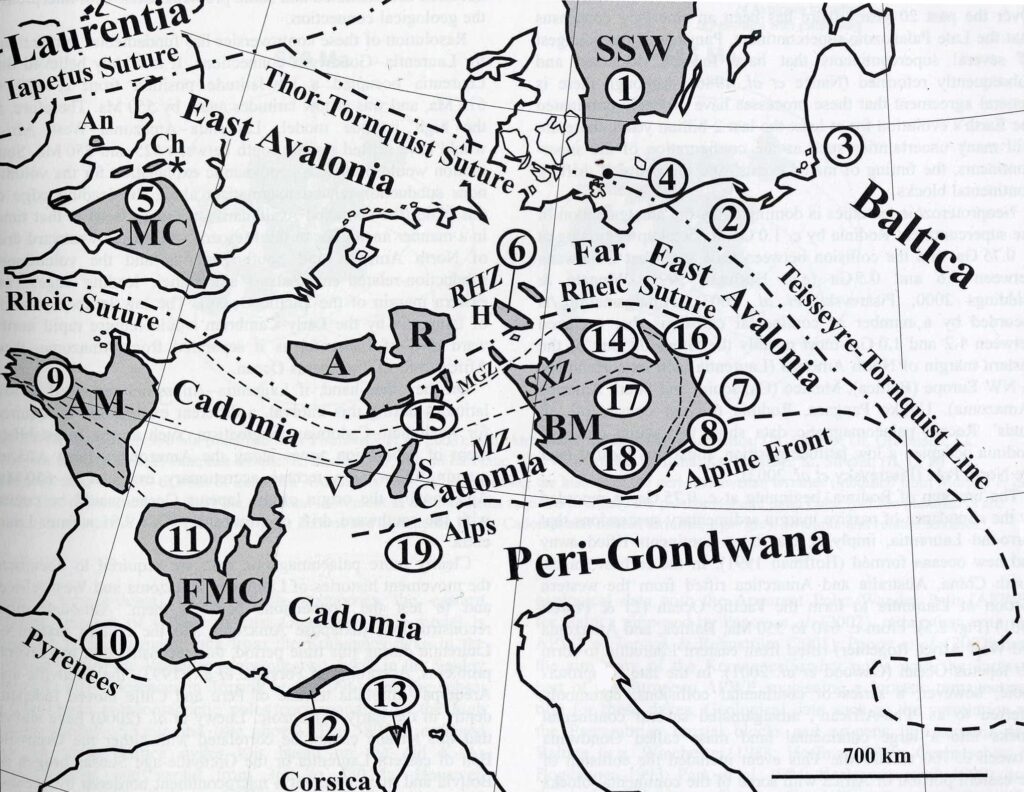
Outcrops
The Armorican Massif is a 65000 km2 plateau in Brittany, Pays de la Loire, and eastern Normandy (NW France.) It is geologically split in 2 domains: the Cadomian Domain and the Hercynian Domain. The Hercynian Domain comprises the southern Armorican Massif and, as suggested by the name, was heavily deformed during the Hercynian (Variscan) orogeny. In contrast, the northern part of the Armorican Massif (the Cadomian Domain) is mostly of Cadomian age – although some overprinting occurred in the Carboniferous (Variscan granites and re-activation of a fault running from Brest to the Sarthe river.)
The Northern Armorican Massif
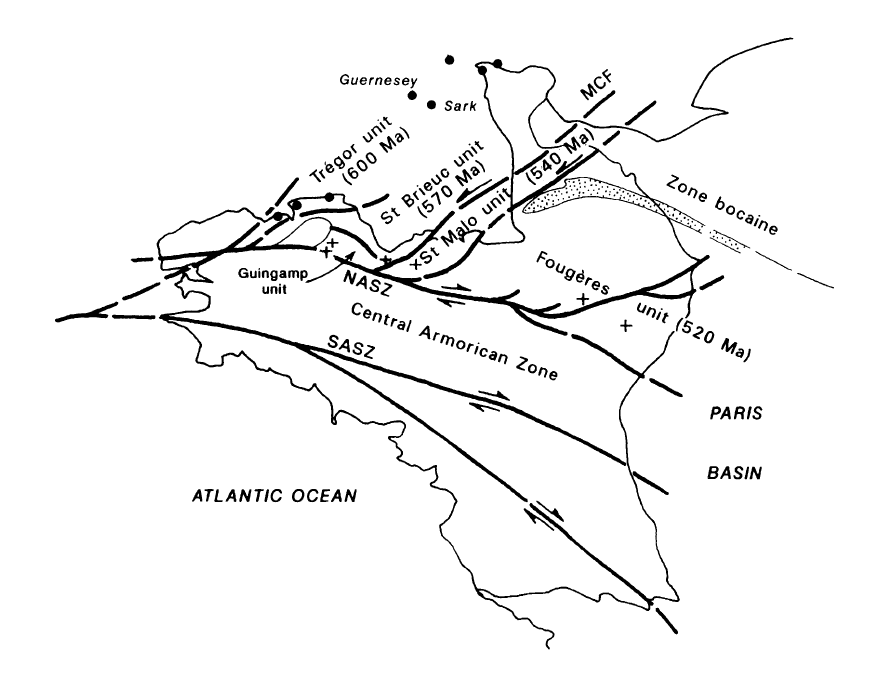
The Northern Armorican Massif can be split into 4 distinct regions, each with its own unique tectonic evolution. These are: the Trégor Terrane, the Saint-Brieuc Terrane, the Saint-Malo Terrane (including the Guingamp Terrane, see Figure 2.1) and the Macellian Terrane (corresponds to the Fougères Unit, see Figure 2.1.) It is important to note that in the literature, nomenclature has been varied, with the terms ‘domain’, ‘terrane’, ‘segment’ and ‘unit’ all being used (D’Lemos et al., 2008, and references within). I will be referring to them as terranes as they are bound together by faults, implying a separate tectonic evolution for each unit (D’Lemos et al., 2008). What follows is an analysis of the lithological components of each terrane and their implications.
Trégor Terrane
The Trégor Terrane (also known as Trégor-La Hague Terrane) is not limited to the mainland, but also includes the islands of Guernsey, Sark, and Alderney. Two distinct phases of activity can be identified here: metamorphosis and later, magmatism. It is also unique, in that rocks from the Icartian basement are found there. These are granite gneisses and have been dated at around 2000 Ma (Samson & D’Lemos, 1998; Inglis et al., 2004.) The gneisses are strongly deformed, however this is not a defining characteristic, so chronometric data is needed to identify those that do belong to the Icartian Basement.
The next (chronological) outcrops we have are metamorphic in nature: deformed and metamorphosed quartz diorites, tonalites, granodiorites and granites are found near La Hague (D’Lemos et al., 2008, and references therein.) The metamorphism, according to Inglis et al. (2004), peaked at around 618 Ma (700 – 800 °C, 8 – 9 kbar.) The metamorphism was followed by intrusions, the main one occurring at 615 Ma (Samson & D’Lemos, 1998) and generally waning over time until their stop at 608 Ma. Cooling then followed.
Graviou and Auvray (1990) suggested a subduction model for the origin of the magmatism, as this would fit with the evolution outlined above. More recent geochemical analyses (e.g. Samson et al., 2003) support this.
The second phase of magmatism occurred around 580 Ma. The intrusions are post-tectonic, as they are not deformed, even within the host rock. They are mostly diorites and granites, but some gabbros are also found. Emplacement occurred during a time of localised deformation and light metamorphosis (greenschist facies) at mid to upper crustal levels.
Moving southward from the Trégor Terrane, we come to the Saint-Brieuc Terrane. Separating the two is the Trégor Fault.
Saint-Brieuc Terrane
A textbook summary of the Saint-Brieuc Terrane would say that it consists of Brioverian sediments deposited on the Penthièvre Complex. The ‘Brioverian’ is a local Neoproterozoic stratigraphic unit spanning from 670 to 540 Ma. It applies only when referring to the Armorican Massif (and related formations such as Jersey, which is generally viewed as belonging to the Saint-Brieuc Terrane,) and is divided in 2 parts: the Lower Brioverian (670 – 590 Ma) and the Upper Brioverian (590 – 540 Ma). The Lower Brioverian generally corresponds to the major magmatic phase and the Lower Brioverian to the sedimentary phase.
The so-called Penthièvre Complex consists of deformed and metamorphosed plutonic rocks. Their cooling ages range from 754 Ma (Samson et al., 2003) to 625 Ma (Nagy et al., 2002). According to Samson et al. (2003), the rocks are not contaminated by the Icartian Basement. Geochemically, the Penthièvre Complex can be seen as being derived from the mantle, which underwent anatexis.
The afore-mentioned Brioverian sediments lie unconformably on the Penthièvre Complex. Around the Bay of Saint Brieuc, the lowest part of the sequence can be seen: it consists of submarine volcanics. These can be traced throughout the region, although their consistencies vary. Around Coutances, they range from basaltic to rhyolitic and contain many pyroclastic ejecta (Dupret et al., 1985.) Around the Bay of Saint-Brieuc, the volcanism is tholeiitic, i.e. typical for an extensional environment. Compare this with the Trégor Terrane, which is generally agreed to have been a subduction zone.
West of the bay, the volcanic succession shifts to a turbiditic sequence (sandstones and siltstones) (Strachan & Roach, 1990). This sequence can be correlated in Jersey (the Jersey Shale Formation), explaining why Jersey is grouped together with the Saint-Brieuc Terrane. Clasts within the turbidites point to a volcanic source.
Also around the bay, we have later magmatic intrusions. These are unrelated to the Trégor Terrane intrusions, despite the similar time range (600 to 570 Ma; Nagy et al., 2002), as their εNd-values differ (Trégor has positive values, whereas these ones are negative.)
Moving southwards, we get to the Saint-Malo Terrane. Separating the two is the Fresnaye Shear Zone (FSZ.)
Saint-Malo Terrane
The main features of the Saint-Malo Terrane are migmatites. There are 3 main belts (D’Lemos et al., 2008, and references therein) consisting of metatexites and diatexites. Partial melting occurred at 650 – 700 °C and 3 – 4 kbar (Ballèvre et al., 2001), corresponding to relatively shallow crustal melting (9 – 12 km). The migmatites are all descended from Brioverian sediments (D’Lemos et al., 2008). U-Pb dating by Peucat (1986) set the anatexis time at ~540 Ma.
Classically grouped separately, the Guingamp Unit is now seen as belonging to the same terrane as Saint-Malo. It contains migmatites formed under similar temperatures and at the same time, however the pressure was significantly higher during their formation, being between 5 and 7 kbar (Ballèvre et al., 2001). A possible explanation suggested by Ballèvre et al. (2001) and, earlier on, by Hébert (1995) is that there were 2 separate periods of anatexis, with both being triggered by crustal thickening.
South of the Saint-Malo terrane lies the Mancellian Terrane, the two separated by the Plouer-Cancale Shear Zone.
Mancellian Terrane
Often referred to as the Fougères Unit, the Mancellian Terrane is dominated by a batholith (the aptly-named Mancellian Batholith) intruding into Brioverian sediments. The sediments are turbidites and submarine fans, including volcanic, metamorphic and plutonic clasts (as well as rarer black cherts.)
The Mancellian Batholith consists of numerous intruded aluminium-rich plutons. Their emplacement took place in the upper crust (maximum 6 km; Ballèvre et al., 2001), as indicated by the contact aureoles. Restites are commonly found near the contact aureoles. Geochemically, the Mancellian Batholith is consistent with melted metasedimentary crust, which also agrees with the restites. At the time of this writing (January 2009), no high-precision dating of the batholith had yet been conducted, however nearby granites (e.g. Vire Granites) have been dated at 547 to 542 Ma (Pasteels & Doré, 1982) and this is seen as a reasonable estimate for the crytallisation of the batholith.
Evolution of the Armorican Massif
Having detailed the lithological characteristics of each unit, it is now necessary to compare them directly. Doing so exposes why the different units are referred to as ‘terranes’: their evolutions are different. The following table (Fig 2.2), taken from The Geology of Central Europe, is a perfect timeline of the different events that happened in the different terranes, and parroting it would not benefit anyone. Instead, I will use it as a base and expand on the terrane theory for the North Armorican Massif.
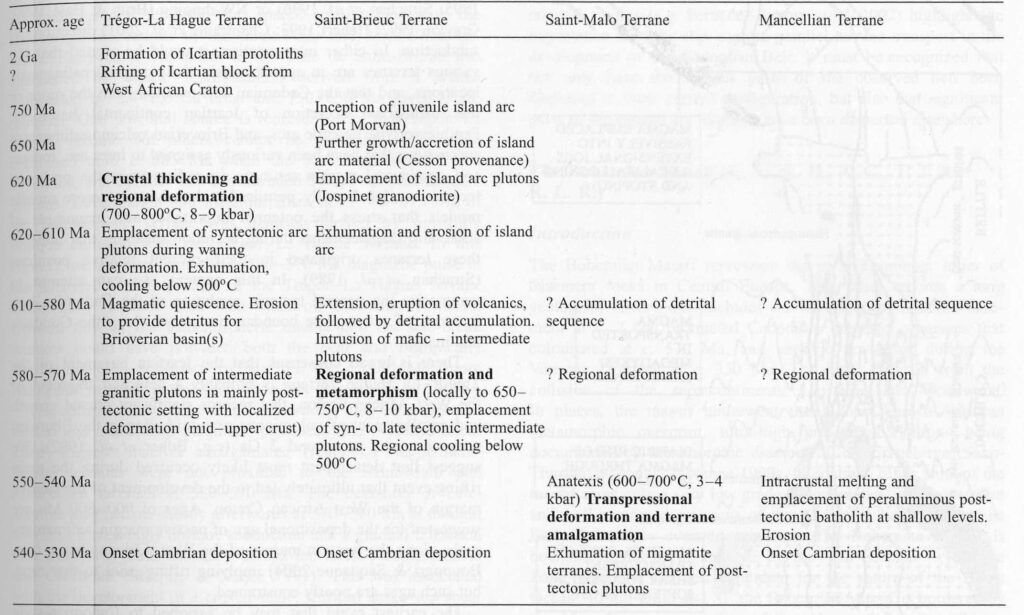
The first thing to note is the difference in plutons between the Saint-Malo Terrane and the Saint-Brieuc Terrane. The latter’s plutons come from the mantle, while the former consists of migmatitic granitoids. As such, the boundary between these two units (the FSZ) is clearly important.
The Trégor-La Hague and Saint-Brieuc terranes were both intruded by plutons between 580 and 570 Ma, pointing to their proximity and possible collision. However, it gets more complicated when looking at the Saint-Malo and Saint-Brieuc terranes. Here, opinions differ as to whether they belong to two different terranes (e.g. Ballèvre et al., 2001) or represent different depths of the same terrane (e.g. Brown, 1995). Proponents of the same terrane theory cite the fact that parts of the Mancellian Batholith are geochemically very similar to the Saint-Malo migmatites (specifically, the leucogranites of the Guingamp Unit.) However, leucogranites are not so common in the Mancellian Batholith, so saying that it is derived from the partial melting that occurred in the Saint-Malo/Guingamp Unit is far-fetched. Clearly, more work is needed in order to conclusively determine the relationship between these two terranes.
The Cadomian Orogeny
Given the lack of evidence for the direction of the subduction and the conflicts about the paleogeographic locations of the terranes, the only way to reconstruct the formation of the Northern Armorican Massif (and consequently, the development of the Cadomian Orogeny) is through theory.
Ballèvre et al. (2001) states that the modern-day location of the terranes reflect their paleogeographical location.
The oldest rocks found are the 2000 Ma Icartian Basement outcrops in the Trégor-La Hague Terrane. Given their age, it is fairly obvious that they did not originate due to Cadomian tectonic processes. The general consensus is that the basement is a part of the West African Craton that rifted away during an earlier orogeny. The first event that can safely be assigned to Cadomian tectonics is the formation of an island arc, as seen in the Penthièvre Complex in the Saint-Brieuc Terrane. The overlying Brioverian sediments over the next 100 Ma suggest the growth of this island arc, pointing to subduction.
The first collision most probably happened at 620 Ma, as we have evidence of crustal thickening in the Trégor-La Hague Terrane. What land mass it collided with is not known. We have evidence for subduction after this event. The magmas derived from this subduction vary: in the Trégor-La Hague Terrane, they are contaminated by the Icartian Basement, while at the Saint-Brieuc Terrane, they are ‘clean’. This may be of paleogeographic importance, if we remember that the Cadomian Orogeny is part of the Pan-African Orogeny, in that it might tell us just how big of a role strike-slip played in the positioning of the terranes.
As of 610 Ma, Brioverian volcanic and sedimentary accumulations began in the Saint-Brieuc Terrane. Whether this was a fore-arc or a back-arc basins cannot be said (again due to paleogeographical uncertainties.) The basin was inverted at 585 Ma, putting a stop to the sedimentation, and in the next 10 Ma, was intruded and deformed by plutons. This can mainly be seen in the Saint-Brieuc Terrane, and it is most plausible that it was caused by a collision with the Trégor-La Hague Terrane, a theory further supported by increased magmatic activity in the Trégor-La Hague Terrane at around the same time, which can be explained by slab break-off.
To summarise the paleogeography at 580 Ma, we can definitely say that the Trégor-La Hague and Saint-Brieuc terranes are close to each other and have probably collided together, and they were both not as close to the Saint-Malo and Mancellian terranes, whose positions are more or less unknown (as mentioned at the end of Section 2, it is not even known whether they are two different terranes to begin with.)
A geochemical change as of 570 Ma points to increased subduction and the formation of a transform fault (Murphy & Nance, 1991). Along with this, a shear zone and fault system developed, which led to the collision of the Saint-Malo and Mancellian terranes (or the one terrane, see end of Section 2) with the already-amalgamated Trégor-La Hague and Saint-Brieuc terranes, thus resulting in the Cadomian Orogen.
Looking at Figure 2.2, we see that more magmatism occurs in the Saint-Malo and Mancellian terranes afterwards (550 Ma onwards.) The reason for this is are as of yet not explained by any model.
Figure 3.1 is a good graphical representation of the Northern Armorican Massif’s evolution.
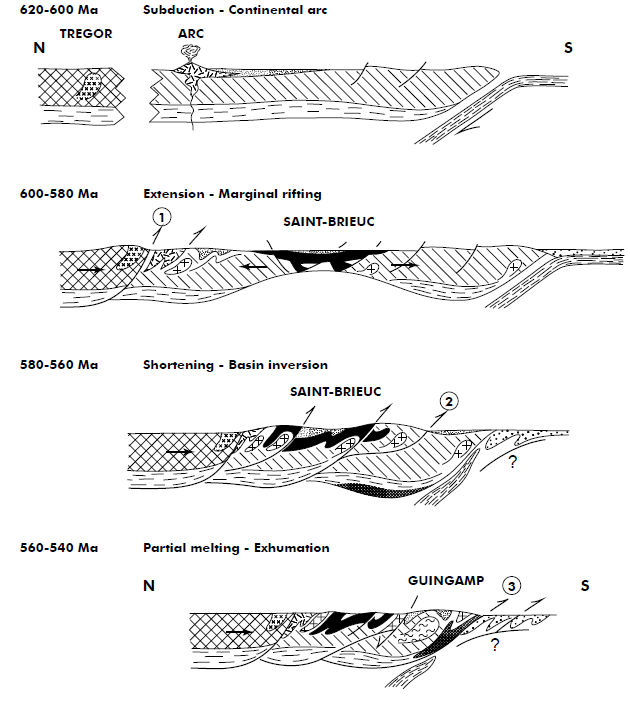
Summary
This is merely a summary of the events detailed above, with more of an emphasis on the actual Cadomian Orogeny and not the Northern Armorican Massif.
The Cadomian Orogeny happened between 750 and 550 Ma at the northwest margin of Gondwana, at the periphery of the West African Craton. Subduction led to the formation of numerous island arcs between 750 Ma and 650 Ma, which collided at 620 Ma, and continued subducting. At 610 Ma, a volcanic basin formed, which then went on to become inversed, deformed, intruded and metamorphosed between 585 and 570 Ma. A switch to a transform margin, as well as the development of strike slip faults and shear zones resulted in the formation of several back-arc basins. A collision with the West African Craton/Gondwana led to the closure of the basins and the formation of a thrust-and-fold belt (i.e. the Cadomian Orogen.)
References
Ballèvre M, Le Goff E. & Hébert R. 2001. The tectonothermal evolution of the Cadomian belt of northern Brittany, France: a Neoproterozoic volcanic arc. Tectonophysics, 331, 19-43.
Brown M. 1995. L’évolution géodynamique à la fin du Précambrien du segment armoricain de la chaîne cadomienne (France) : déformation d’une marge continentale active pendant une convergence vers le sud-ouest et une subduction d’un relief bathymétrique. Géologie de la France, 3, 3-22.
D’Lemos RS, Samson SD & Strachan RA. 2008. The Northern Armorican Massif. In Chapter 3: Linneman U (co-ordinator). Cadomian Tectonics. In: McCann T. 2008. The Geology of Central Europe, Volume 1, Geological Society, London.
Dupret L, Le Gall J, Doré F, Gatinot F & Dissler E. 1985. Les spilites de Vassy (Calvados), témoin d’un volcanisme sous-marin tholéiitique et distensif dans la sédimentation du Briovérien supérieur (NE du Massif armoricain). Comptes rendus de l’Académie des sciences, 300, 687-692.
Graviou P & Auvray B. 1990. Late Precambrian M-type granitoid genesis in the Cadomian belt of NW France. Geological Society, London, Special Publications, 51, 231-244.
Hébert R. 1995. Evidence for multiple high-T metamorphism and regional migmatization within the Cadomian belt of northern Brittany, France. Journal of the Geological Society, 152, 213-216.
Inglis JD, Samson SD, D’Lemos RS & Hamilton M. 2004. U–Pb geochronological constraints on the tectonothermal evolution of the Paleoproterozoic basement of Cadomia, La Hague, NW France. Precambrian Research, 134, 293-315.
Murphy JB & Nance RD. 1991. Supercontinent model for the contrasting character of Late Proerozoic orogenic belts. Geology, 19, 469-472.
Nagy EA, Samson SD & D’Lemos RS. 2002. U–Pb geochronological constraints on the timing of Brioverian sedimentation and regional deformation in the St. Brieuc region of the Neoproterozoic Cadomian orogen, northern France. Precambrian Research, 116, 1-17.
Pasteels P & Doré F. 1982. Age of the Vire-Carolles granite. In: Odin G. Numerical dating in stratigraphy. John Wiley & Sons, London, UK.
Peucat JJ. 1986. Behaviour of Rb-Sr whole rock and U-Pb zircon systems during partial melting as shown in migmatitic gneisses from the St Malo Massif, NE Brittany, France. Journal of the Geological Society, 143, 875-885.
Strachan RA & Roach RA. 1990. Tectonic evolution of the Cadomian belt north Brittany. Geological Society, London, Special Publications, 51, 133-150.
Samson JD & D’Lemos RS. 1998. U–Pb geochronology and Sm–Nd isotopic composition of Proterozoic gneisses,Channel Islands, UK. Journal of the Geological Society, 155, 609-618.
Samson SD, D’Lemos RS, Blichert-Toft J. & Vervoort J. 2003. U-Pb geochronology and Hf-Nd isotope compositions of the oldest Neoproterozoic crust within the Cadomian orogen: new evidence for a unique juvenile terrane. Earth and Planetary Science Letters, 208, 164-180.